The Future of Load Balancing: Adapting to Renewable Energy Shifts by 2030
Europe aims to integrate more clean energy by 2030, but faces a big challenge: what happens when there is too much or too little electricity? This article takes a close look at how Europe in total can keep energy supplies reliable as we transition to fluctuating renewable sources such as wind and solar and take Austria as a deep dive example.
To keep the electrical grid stable and balanced between the supply and demand while meeting our ambitious climate targets, we need smart technology to store surplus renewable power to be utilized at a different point of time and we need smart strategies to manage energy consumption and collective responsibilities through demand side management. Securing the stability of the electrical grids is all about balancing periods of low power supply with high demand and vice versa. This article shows that everyone can help by simply using electricity wisely, like charging electric cars at certain times. This helps to keep the lights on and makes sure we’re not wasting energy, which is good for the planet. Looking at both high-tech solutions and new ways to use energy more intelligently, we set out what needs to be invested to secure a future where energy is both green and always available when we need it. The following are the main key takeaways from this article.
- Renewable Energy Revolution: Global efforts are underway to transition towards sustainable energy sources such as wind and solar power. This imperative shift aims to combat climate change and ensure a greener future for generations to come.
- Supply-Demand Dynamics: With the increasing electrification of sectors like transportation and heating, Europe in general and Austria as an example anticipates a surge in power demand. Concurrently, the integration of intermittent renewables necessitates a delicate balance between supply and demand to maintain grid stability and resilient.
- Load Balancing Essentials: Load balancing techniques are pivotal in ensuring the equilibrium between electricity supply and demand. These strategies are like juggling, mitigate imbalances, and averting disruptions in the power grid.
- Technology Toolbox: Various technologies, such as pumped hydro storage and lithium-ion batteries, offer solutions for effective load balancing. Selecting the appropriate technology hinges on the use case and various other factors such as geographical feasibility and economic viability.
- Collective Responsibility: Apart from using technology, it’s important for society to get involved by managing how much energy they use and being careful about it. This helps a lot in balancing the load effectively. By collectively embracing energy efficiency measures, we contribute to a resilient and sustainable energy landscape. Actively implementing Demand Side Response practices might reduce the peak load up to 22% in 2030. This as a return might cover the gap between the supply and demand, ensure further utilization of renewable energy, and avoid investing in expensive load balancing technologies.
The transition towards renewable energy sources is redefining the energy landscapes of various countries around the world. The ongoing climate change measures are important and urgently needed to assure the preservation of our planet’s delicate ecosystems, mitigate the devastating impacts of global warming, and secure a sustainable future for generations to come. This article synthesizes the projected energy trends, the critical role of demand-side management, and the integration of various load-balancing technologies. By understanding these components, stakeholders can better prepare for the shifting dynamics of the future energy market.
Europe’s energy sector is undergoing significant transformation, with a steady increase in power demand anticipated in the next decade. The electrification of transport and the production of green hydrogen, as well as the utilization of heat pumps are primary drivers, pushing the demand higher. However, they can also be utilized to provide flexibility to the grid. Concurrently, more than 650 gigawatts peak of intermittent renewable power, predominantly from wind and solar sources, are expected to be installed in Europe, reshaping the energy supply structure. However, the phase-out of coal and nuclear assets brings to the forefront the necessity for dependable load-balancing technologies. These technologies play a crucial role in managing the diurnal and seasonal gap between peak load demand and available power capacity, while optimizing the utilization of renewable energy and ensuring grid stability.
To grasp the necessity of load balancing, consider the depiction in Figure 1, which presents a schematic diagram of load and supply curves within a net-zero scenario driven solely by renewable energy sources. Notably, regions denoted by “A” exhibit an excess of renewable power, necessitating curtailment to maintain grid stability, or alternatively, balancing the demand with supply through downward load balancing measures including demand side management by decreasing the demand. Conversely, regions labelled as “B” face a shortage of power supply, indicating the need for either augmented generation capacity or demand reduction to achieve upward load balancing.
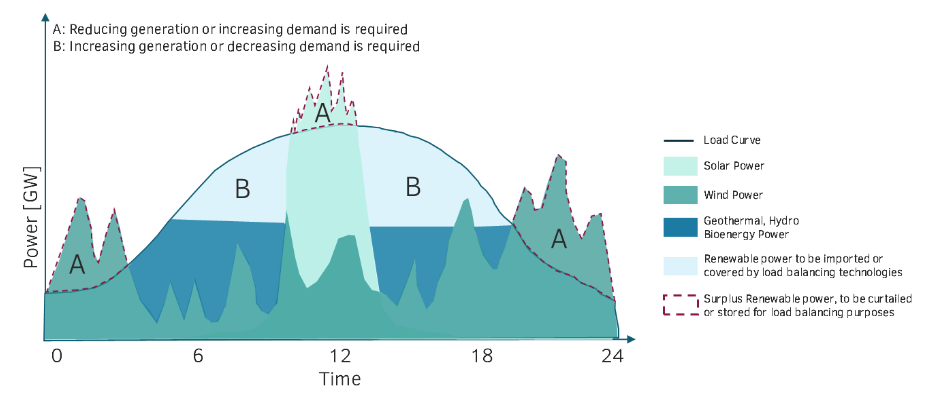
Load Balancing Technologies & the Role of Demand Side Management
However, which technologies of load balancing are available?
Load balancing technologies are represented by the various energy storage systems, out of which various commercially available solutions already exist. Table 1 shows and clarifies some of the most readily accessible and significant technologies.
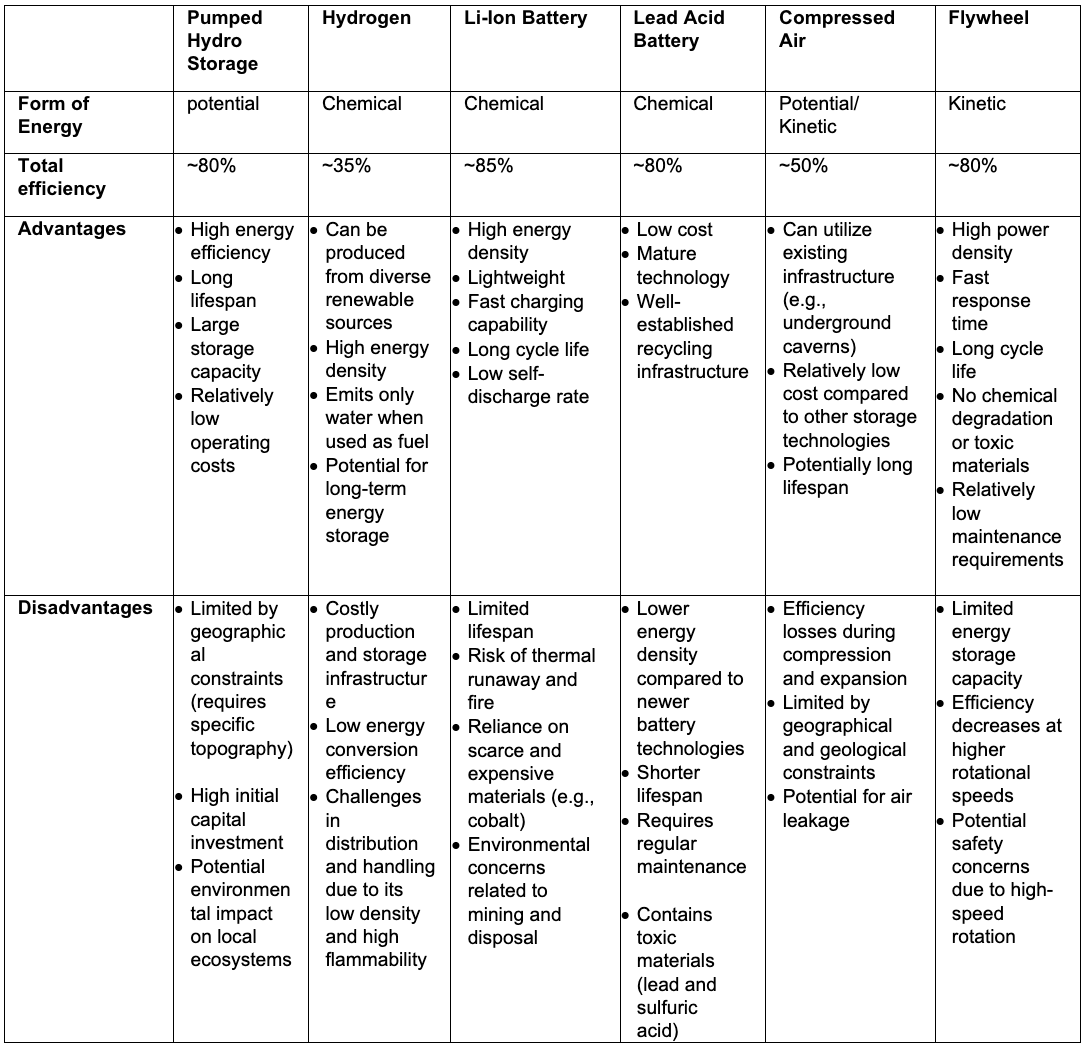
Choosing one of these technologies for load balancing is not that simple and depends on various factors such as the accessibility of the geographical location, the capital cost, the gap between peak load and power generation, how often this peak load occurs and for how long, and many more factors. Normally, multiple technologies are used in order to ensure covering the whole load balancing demand spectrum, especially when considering the technical, environmental, economic, and geographical limitations of the various technologies.
For example, in Austria 75% of the hydropower potential is already exploited, considered one of the leading countries worldwide, while covering 57% of its national power generation. Exploiting the rest of 25% has, however, various economic, geographic, and environmental concerns since it’s mainly deployed in the west of Austria. Hence, further investment may be required to upgrade the grid and connect the west of Austria to the east of Austria to enable balanced power generation and supply.
Europe faces the potential of encountering a deficit in generation capacity of approximately 60 GW with a peak demand of ~750 GW by 2030, necessitating a combination of actions beyond the swift growth of renewable energy sources to address this shortfall. In order to fill this gap Europe needs to take a bundle of measures that would secure the power supply including load balancing technologies and further approaches such as the demand side management.
Considering Austria as a case in point within Europe, situated centrally and aimed to reach climate neutrality by generating 100% renewable power in 2030, with a population comprising just 2% of the EU27 and an energy demand constituting less than 1% of the EU27 total, the forecasted power deficit for 2030 is estimated at around 6 GW, equivalent to approximately 10% of the EU27 shortfall. This gap comes along the Austrian initiative to further increase the renewable power generation.
However, what could be the investment costs for various load balancing technologies to secure the stability of the grid and close the power gap? Table 2 illustrates the estimated investment capital costs for the technologies shown in Table 1 per unit and the total costs to cover the 60 GW gap in Europe and the 6 GW in Austria. For comparison the table includes the investment costs of a gas power plant as a reference.
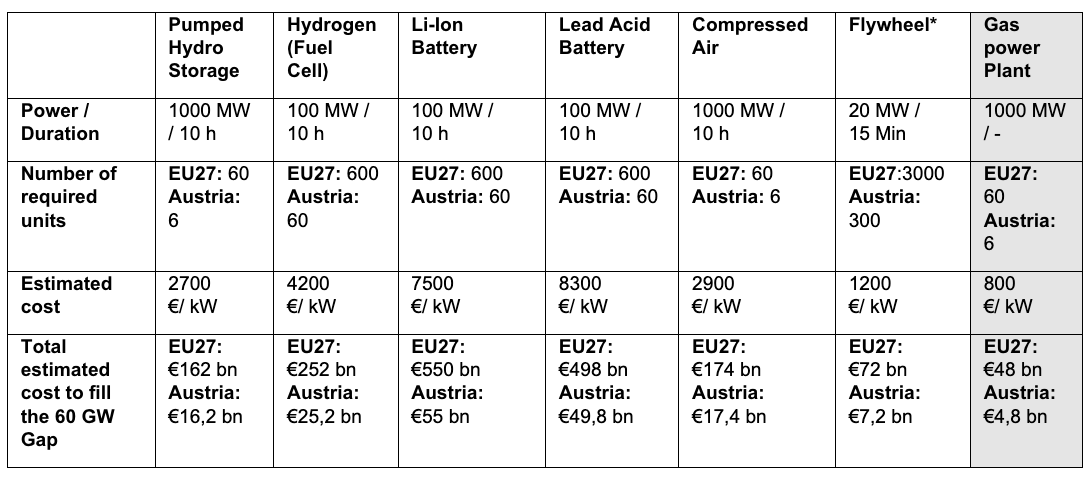
Focusing on Austria, the expected 6 GW gap in 2030 between supply and demand underscores the complexity of Austria’s energy transition. Addressing this disparity requires significant investments in load balancing technologies and demand-side management.
Investing in load-balancing technologies requires a holistic approach, including financial, infrastructural, and regulatory considerations. Repowering existing installations could be a game-changer, as exemplified by the second-life batteries. Moreover, streamlined permitting processes and public engagement can accelerate the deployment of necessary infrastructure, enable the demand side management, hence, ultimately closing the gap between peak load demands and renewable energy availability.
Demand-side management emerges as a pivotal strategy in this evolving energy landscape. By implementing flexible tariffs and encouraging technologies like vehicle-to-grid systems, peak energy demands can be shaved, thereby reducing the gap between demand and supply, if not covering it all. This approach not only enhances grid stability but also paves the way for a more sustainable energy future, harnessing the full potential of renewable sources.
Envisaged demand response incentives spanning various sectors hold the potential to substantially decrease peak load in EU27 by around 22% via upward load balancing and increase the load by up to 17% when it is required to balance between the supply and demand of electricity through downward load balancing (check Figure 1 for better understanding). Figure 2 illustrates the potential available flexible power through demand side management of various technologies in EU27 in 2030 referring to its peak demand.
To put the scale of the 6 GW gap in Austria and the importance of demand side management into perspective, envision this: it would take the collective power of 30,000 fully charged Tesla Model 3 vehicles, each boasting a rated output power of approximately 200 kW, to effectively bridge this gap.
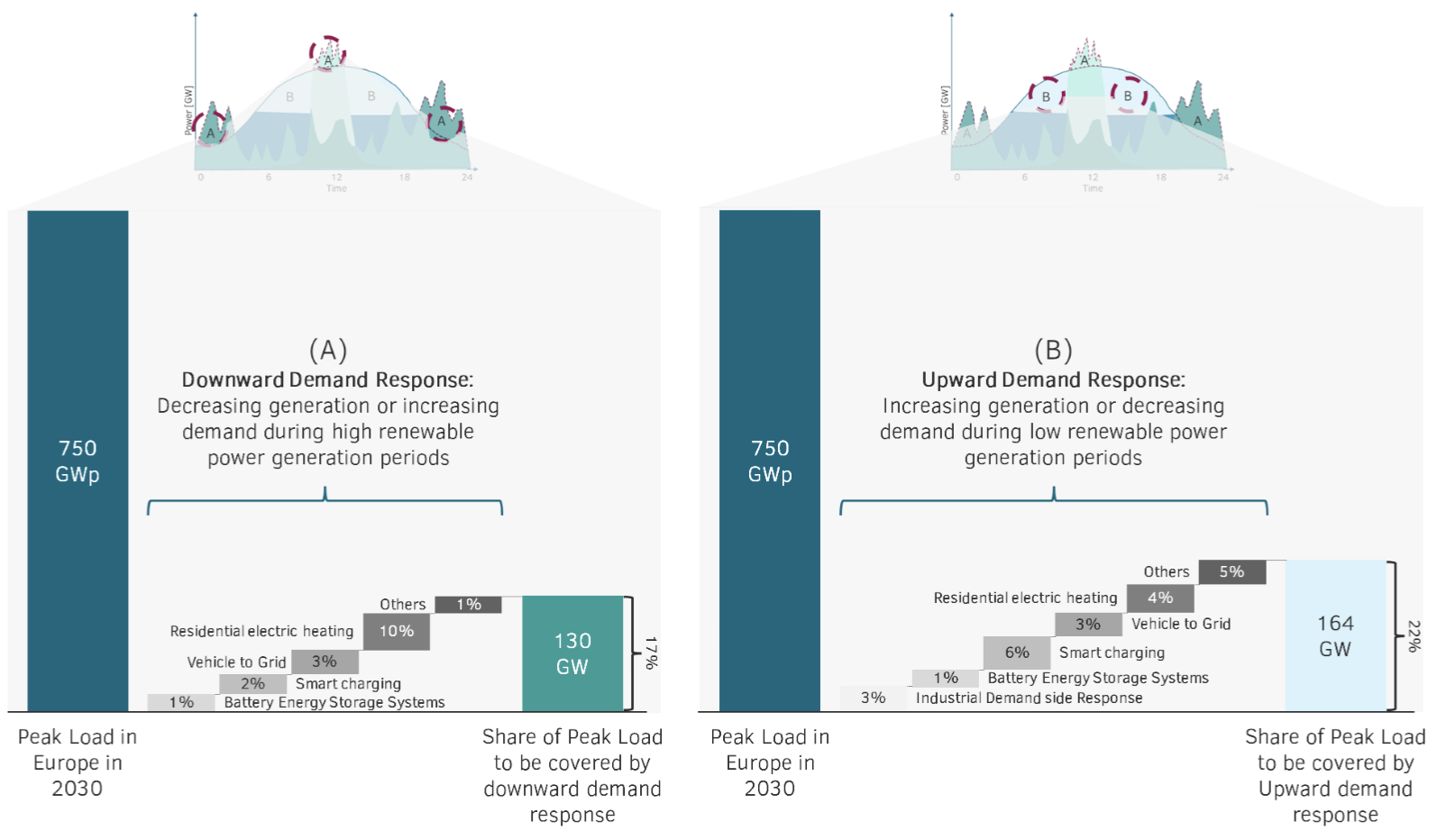
As a conclusion, the journey towards a renewable-driven future is fraught with challenges, particularly in maintaining grid stability amid fluctuating energy supplies. Strategic investments in load-balancing technologies, coupled with innovative demand-side management, are paramount for Europe in general and Austria specifically to meet its 2030 energy objectives. As the landscape evolves, so too, must our approaches to ensuring a balanced, resilient, and sustainable energy grid.
Get in touch with us:
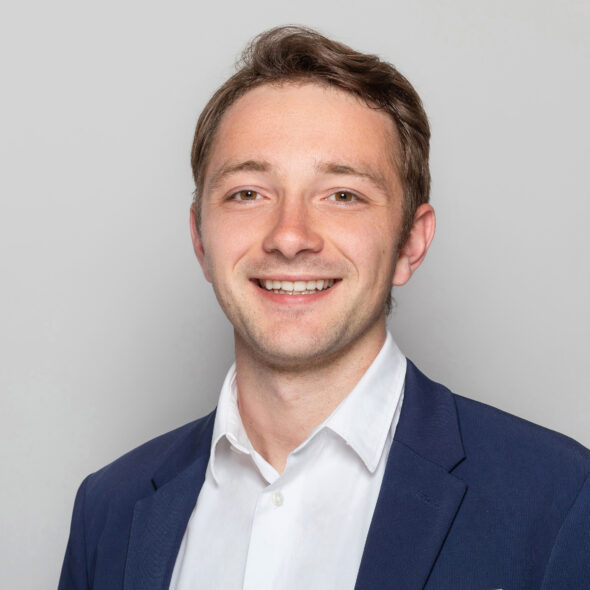
David Schneiderbauer
Senior Manager